About the program
One of the key open issues in high energy astrophysics is that there is no agreement on the origin and the energy source of relativistic jets launched from compact objects. In particular, we still do not understand the relationship between the properties of the accretion flow (geometry, mass inflow rate, radiative power), the spin parameter of the compact object, and the properties of the relativistic jet (kinetic power, mass outflow rate, time variability). Determining in what circumstances jets arise as a by-product of accretion, and quantifying how much power they inject into the surrounding medium, are problems of broad astrophysical importance (e.g., jet feedback can affect other astrophysical processes, such as star formation and galaxy evolution; [1, 2]). A crucial step towards understanding the inner workings of these jets is characterizing jet properties and how they evolve. Here we will focus in particular on black hole jets, as we want to quantify how their properties scale from stellar-mass systems to the most massive nuclear black holes.
Black Hole X-ray Binaries
Stellar mass black hole systems in our Galaxy, known as black hole X-ray binaries (BHXBs), are ideal laboratories for studying the jet/accretion relationship, as they are numerous, close in proximity, display a wide range of accretion and jet launching environments, and evolve on human timescales. BHXBs contain a black hole accreting matter from a companion star, where a portion of the accreted material can be ejected in the form of a relativistic jet (see Figure 1). The majority of these systems are transient, evolving from periods of minimal activity into a bright outbursting state on timescales of days to months [3]. Jet emission in BHXBs is produced as a result of synchrotron emission [4, 5], and dominates at longer wavelengths (radio, sub-mm, IR; [6, 7, 8]), while emission from the accretion flow dominates at shorter wavelengths (optical, UV, X-ray; [9]).
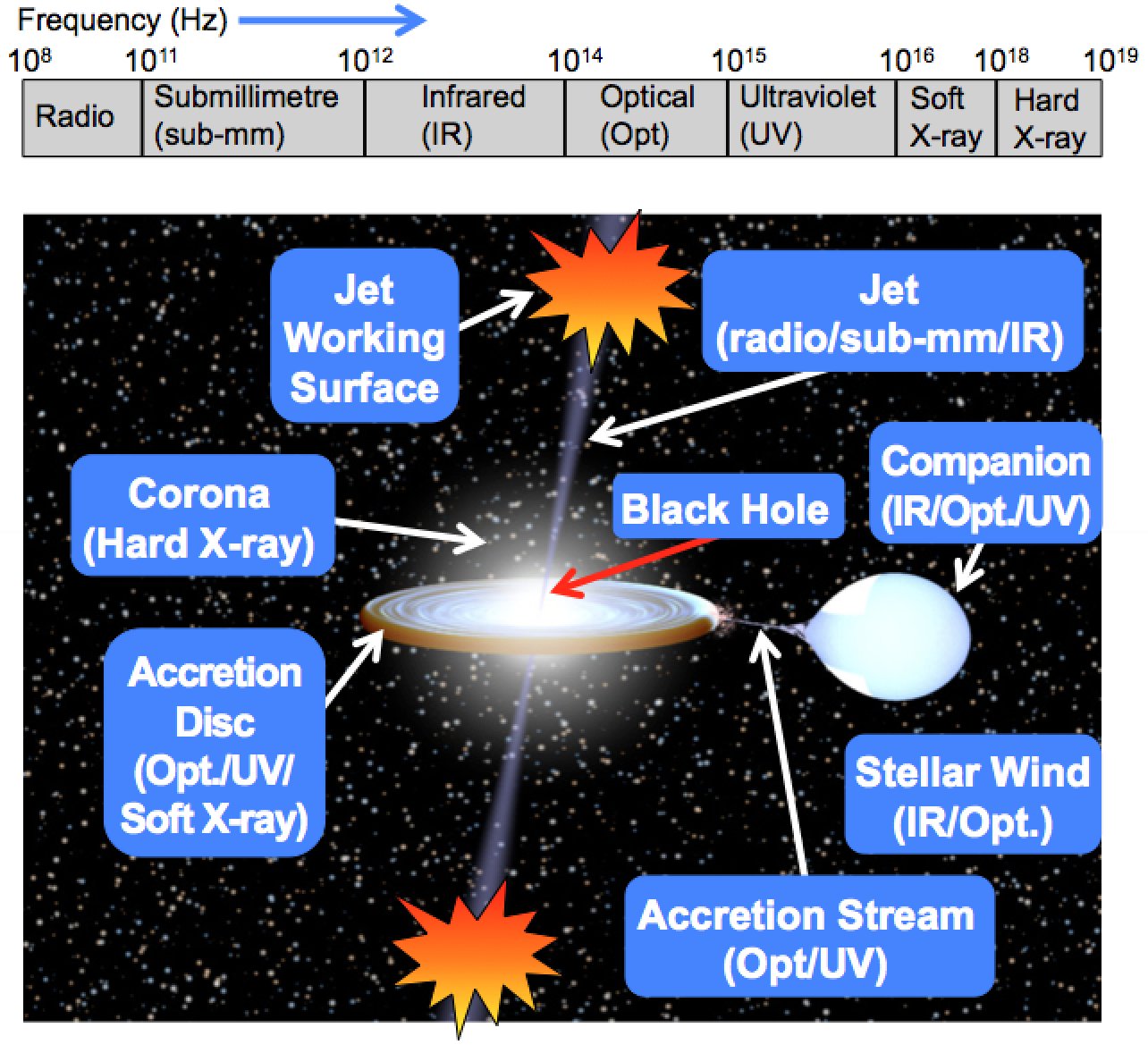
The sub-mm bands uniquely allow us to isolate radiation produced by the jet from radiation produced in the accretion flow near the compact object, where the jet is launched and particles are first accelerated to high energies. However, despite the importance of the sub-mm wavebands, there are only a handful of X-ray binary studies in this regime, the majority of which are purely photometric studies. By delving into the time domain and polarimetric properties of sub-mm jet emission we have the opportunity to accurately measure key jet properties (e.g., geometry, speed, power; e.g., see Figures 2a and b, and [12]), understand what mechanisms drive changes in these properties, and take a step forward in answering the fundamental open questions in jet research (e.g., jet launching and acceleration mechanisms, magnetic field structure).
The PITCH-BLACK Survey
The PITCH-BLACK Survey is a new JCMT Large Program, which will perform SCUBA-2/POL-2 observations of 6 outbursting BHXBs over the next 3 years. With these data, we will sample jet production in a range of different sources and accretion regimes, allowing us to gain crucial insights into jet physics, particle acceleration, and how these processes depend on the accretion flow properties. The three key elements of our strategy to achieve these goals are: i) select black hole jets that vary on short timescales; ii) observe them in an electromagnetic band that maximizes the jet emission component; iii) use polarimetry to understand the physical processes.
Open questions the PITCH-BLACK survey aims to answer:
(a) How are jets launched and accelerated in black hole systems?
(b)What are the initial conditions for the formation of jets in the launching/acceleration region?
(c) What role do black hole mass, spin, and accretion rate play in jet production? How do our results from stellar-mass black holes scale to super-massive black holes?
(d) What factors drive jet evolution during outburst? Does the jet ever become fully quenched during outburst?
(e) What is the origin and structure of the magnetic fields in jets?
(f) How does the energy released by BHXB jets compare to other feedback processes, such as star formation?
References
- [1] Silk, J., & Rees, M., 1998, A&A, 331, L1
- [2] Mirabel, I. F., et al., 2011, A&A, 528, 6
- [3] Tetarenko, B. E., et al., 2016, ApJSS, 222, 15
- [4] Hjellming, R. M., & Johnston, K. J., 1988, ApJ, 328, 600
- [5] Corbel, S., & Fender, R. P., 2002, ApJ, 573, L35
- [6] Fender, R., 2001, MNRAS, 322, 31
- [7] Russell, D.,et al., 2006, MNRAS, 371, 1334
- [8] Tetarenko, A. J., et al., 2015, ApJ, 805, 30
- [9] Done, C., et al., 2007, A&ARv, 15, 1
- [10] Tetarenko, A. J., et al., 2017, MNRAS, 469, 3141
- [11] Miller-Jones, J. C. A., et al., 2019, Nature, 569, 374
- [12] Tetarenko, A. J., et al., 2019, MNRAS, 484, 2987